Concrete boasts an annual production of 3.8 billion cubic metres making it the cornerstone of construction. Its versatility, tensile strength and cost-effectiveness have solidified its position as the most widely used construction material. However, concrete’s susceptibility to cracking undermines its structural integrity, allowing water and harmful substances to corrode reinforcements thereby shortening the lifespan of structures. Traditional repair methods of concrete like epoxy injections or patching that have been used to close cracks are often expensive and bad for the environment. In this article we will discuss self healing concrete, a new technology that is solving all these problems;
The Mechanisms
Self-healing concrete technologies can be broadly classified into autogenous and autonomous methods. Autogenous healing relies on natural hydration of unreacted cement particles and calcium carbonate precipitation and is typically triggered by water exposure. Autonomous healing on the other hand introduces external agents such as polymers, bacteria, encapsulated chemical compounds or superabsorbent polymers (SAPs).
Among these, microbial-induced calcite precipitation (MICP) has garnered significant attention. Bacteria such as Bacillus subtilis and Halobacillus halophilus thrive in concrete’s alkaline environment producing calcium carbonate that seals fissures and restores structural integrity.
Recent advancements in microbial self-healing technologies include genetic modifications to enhance bacterial resilience, integration of additives to optimize metabolic pathways and encapsulation techniques to improve bacteria survival under harsh conditions. For instance, calcium peroxide embedded within bacterial spores facilitates near-complete crack closure within 30 days, demonstrating the potential of microbial self-healing systems. Moreover, advanced metabolic engineering techniques have enabled bacteria to thrive in extreme environments, expanding their applicability to challenging construction conditions.
Encapsulation and Chemical Systems
Encapsulation has emerged as a transformative approach, enabling precise delivery of healing agents when cracks appear. Cement-shell microcapsules have proven particularly effective offering compatibility with concrete’s matrix while protecting healing agents. This method is adaptable to chemical agents like sodium silicate and SAPs which react with environmental triggers such as moisture to seal cracks. Ultrasonic wave-induced triggers represent another breakthrough which allows non-invasive activation of healing processes at precise locations. The use of these triggers has also opened possibilities for targeted repairs in highly complex structures, reducing material waste and optimizing repair strategies.
Chemical-based self-healing methods have also advanced significantly. Agents such as sodium silicate and calcium sulfoaluminate react with moisture to form gels or precipitates that seal cracks and restore durability. Superabsorbent polymers not only facilitate crack closure but also retain water for internal curing, enhancing long-term durability. However, challenges persist, including the impact of chemical agents on concrete’s fresh properties, necessitating the use of superplasticizers. Research into optimizing the particle size and distribution of these agents continues to improve their integration into concrete systems without compromising mechanical performance.
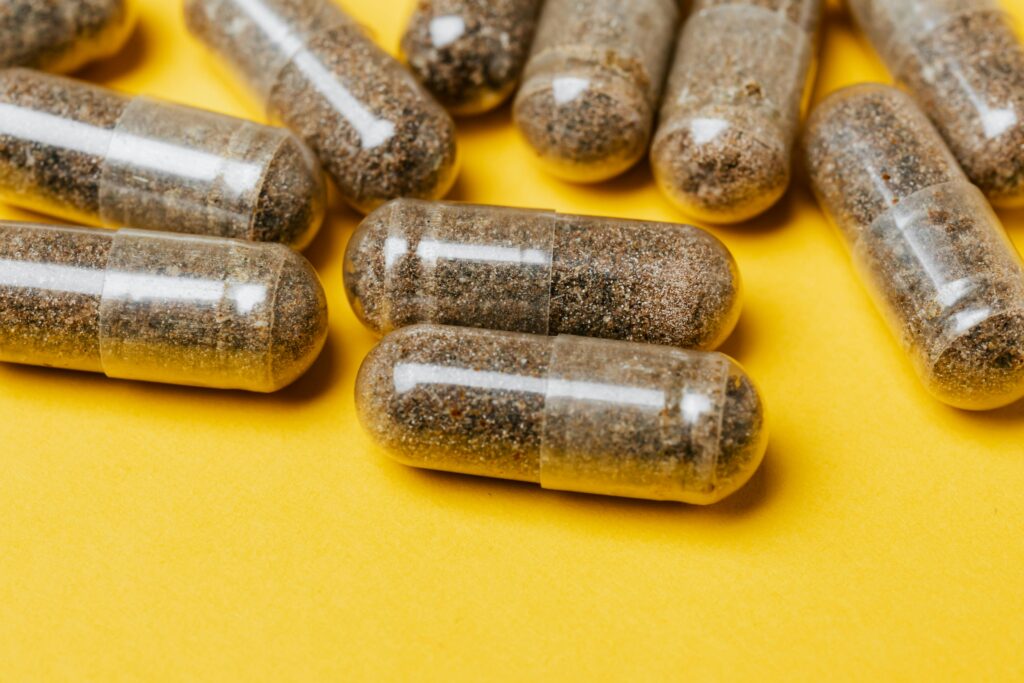
Real-World Applications
Self-healing concrete has shown immense potential across various construction domains:
- Infrastructure and Transportation
Major infrastructure projects, including bridges, tunnels, and highways benefit greatly from self-healing concrete. By autonomously repairing cracks, it prevents water and chloride ingress, significantly extending the service life of structures. The Valleys Highway Project in Wales demonstrated the efficacy of self-healing concrete on a large scale, integrating techniques such as bacterial healing and microcapsules to reduce crack formation and enhance durability. Such projects underline the importance of combining different self-healing techniques to meet the unique demands of large-scale infrastructure.
2. Marine and Coastal Structures
Marine environments impose harsh conditions that accelerate concrete degradation through chloride and sulfate attacks. Bacteria-based systems, such as those incorporating Halobacillus halophilus have proven effective in sealing cracks in submerged and tidal conditions, enhancing the durability of seawalls, piers, and offshore platforms. Additionally, encapsulated agents that withstand extreme salinity have been shown to significantly enhance the longevity of concrete in these settings, offering a reliable solution for marine construction challenges.
3. Industrial and Specialized Constructions
Industrial facilities often face significant mechanical and chemical stresses. Self-healing concrete reduces downtime and enhances durability, eliminating the need for specialized coatings. In nuclear facilities, its ability to seal cracks autonomously minimizes the risk of radiation leakage. Furthermore, the technology’s adaptability to extreme conditions ensures it can be tailored for specialized applications, such as containment vessels for hazardous materials.
4. Sustainable Developments
Aligning with global sustainability goals, self-healing concrete reduces lifecycle costs and CO2 emissions. For instance, Basilisk’s liquid repair system ER7 extended the lifespan of a bus lane at Schiphol Airport by 15 years while cutting lifecycle costs by 33% and CO2 emissions by 90%. Beyond its direct environmental benefits, the material’s longevity contributes to reduced resource consumption and less frequent need for structural interventions, emphasizing its role in sustainable urban development.
5. Water Retaining Structures
Dams, reservoirs, and water purification plants benefit significantly from self-healing concrete which maintains impermeability by sealing cracks quickly. Encapsulation technologies and shape memory polymers have proven particularly effective in these applications. The adoption of these materials has not only improved durability but also reduced maintenance demands, ensuring continuous operation of critical water infrastructure.
CHALLENGES AND FUTURE DIRECTION
Despite its transformative potential, self-healing concrete faces several challenges:
- Material Incompatibility
ome healing agents react with concrete components, adversely affecting setting times and mechanical properties. For instance, calcium oxide can reduce compressive strength and disrupt microbial activity. Optimizing particle sizes and formulations is essential to mitigate these effects. Additionally, ongoing research into bio-compatible additives aims to reduce adverse interactions, paving the way for more seamless integration of healing agents.
2. High Costs
Sophisticated production techniques for encapsulation systems and finely engineered SAPs inflate material costs, limiting adoption in large-scale projects. Streamlining production and scaling up processes could help reduce costs. Moreover, investment in automated manufacturing processes holds promise for making advanced self-healing systems more economically viable in the near future.
3. Environmental Concerns
While self-healing concrete aims to reduce environmental impact, certain materials, such as synthetic carriers and nutrient sources like urea, raise sustainability concerns. Developing bio-based carriers and alternative nutrient systems is crucial to addressing these issues. Efforts to replace synthetic polymers with biodegradable alternatives also show potential for enhancing the environmental compatibility of self-healing systems.
4. Long-Term Durability
Freeze-thaw cycles, chemical exposure, and marine conditions pose challenges to the long-term performance of healing agents. Ensuring bacterial viability and optimizing encapsulation techniques are key research areas. Advances in encapsulation, such as multi-layered protective shells, have improved the survival rates of bacterial agents, but further work is needed to ensure durability over decades.
5. Scalability
Large-scale implementation of self-healing technologies requires overcoming logistical hurdles such as integrating these systems into standard construction processes. Advanced simulation tools and artificial intelligence can aid in designing more efficient self-healing systems. The use of predictive modeling can also optimize placement and quantity of healing agents in structures reducing material use while maintaining performance.
Conclusion
Self-healing concrete represents a shift in construction as we know it by offering solutions to problems that have plagued the construction industry for decades. By addressing these challenges, this technology has the potential to revolutionize infrastructure and create a more sustainable built environment. Expanding its application in diverse environments and refining production methods will be key to unlocking its full potential ovr the years. With collaborative efforts among researchers, industry professionals, and policymakers, self-healing concrete can pave the way for more resilient and eco-friendly infrastructure globally.